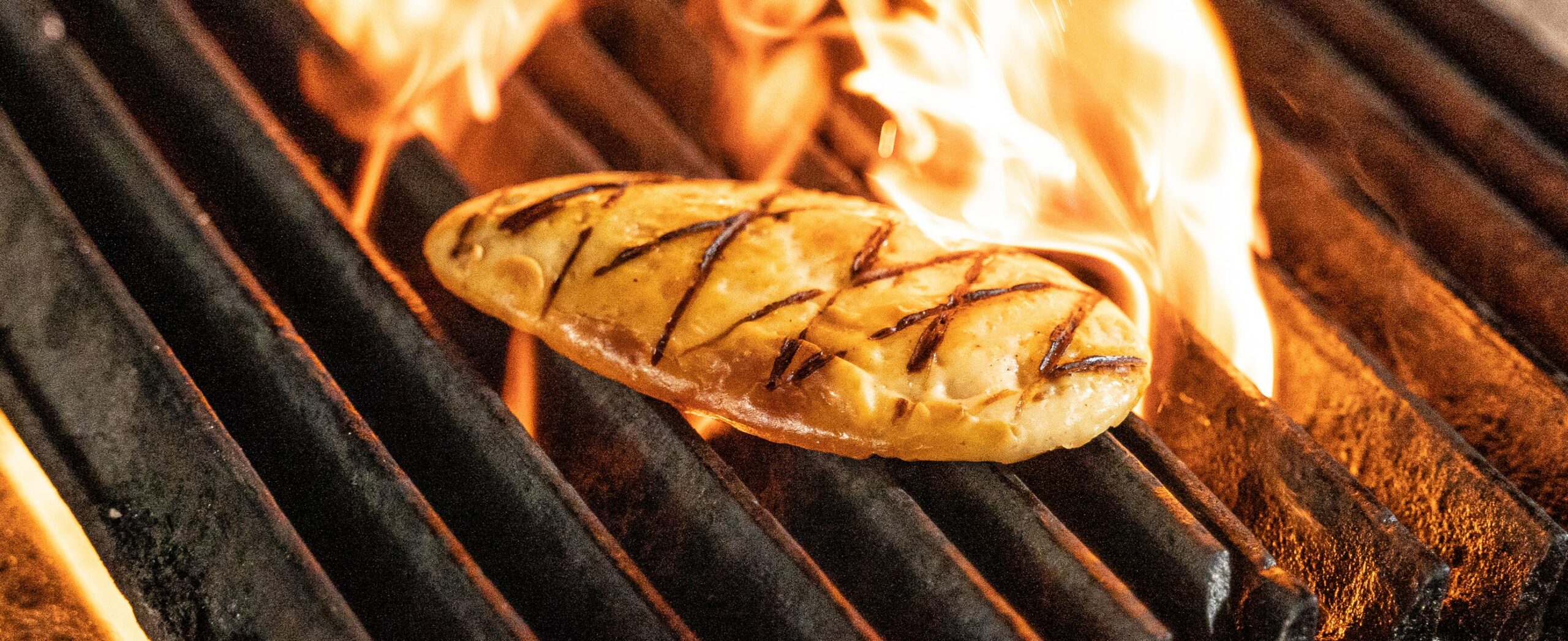
The science of cultivated meat
Learn about the science behind cellular agriculture and the challenges that must be addressed for commercial production.
Introduction to cultivated meat
What is cultivated meat?
Cultivated meat is genuine animal meat (including seafood and organ meats) that is produced by cultivating animal cells directly. This production method eliminates the need to raise and farm animals for food. Cultivated meat is made of the same cell types arranged in the same or similar structure as animal tissues, thus replicating the sensory and nutritional profiles of conventional meat.
Dutch scientist Mark Post unveiled the first cultivated meat burger on live television in 2013. Two years later, the first four cultivated meat companies were founded. The industry has since grown to more than 70 companies on six continents, backed by $450M+ in investments, each aiming to produce cultivated meat products. Dozens more companies have formed to create technology solutions along the value chain.
Decades of accumulated knowledge in cell culture, stem cell biology, tissue engineering, fermentation, and chemical and bioprocess engineering preceded the field of cultivated meat. Hundreds of companies and academic laboratories worldwide are conducting research across these disciplines to establish a new paradigm for manufacturing commodity meat products at industrial scales.
How is cultivated meat made?
The manufacturing process begins with acquiring and banking stem cells from an animal. These cells are then grown in bioreactors (known colloquially as cultivators) at high densities and volumes. Similar to what happens inside an animal’s body, the cells are fed an oxygen-rich cell culture medium made up of basic nutrients such as amino acids, glucose, vitamins, and inorganic salts, and supplemented with proteins and other growth factors.
Changes in the medium composition, often in tandem with cues from a scaffolding structure, trigger immature cells to differentiate into the skeletal muscle, fat, and connective tissues that make up meat. The differentiated cells are then harvested, prepared, and packaged into final products. This process is expected to take between 2-8 weeks, depending on what kind of meat is being cultivated. Some companies are pursuing a similar strategy to create milk and other dairy products.
What are the benefits of cultivated meat?
By nature of its more efficient production process, cultivated meat is expected to have a variety of benefits over conventional animal agriculture. Prospective life cycle assessments indicate that cultivated meat will use significantly less land and water, emit fewer greenhouse gases, and reduce agriculture-related pollution and eutrophication.
According to a 2020 publication in Nature Food, commercial production is expected to occur entirely without antibiotics and is likely to result in fewer incidences of foodborne illnesses due to the lack of exposure risk from enteric pathogens.
Over the next few decades, cultivated meat and other alternative proteins are predicted to take significant market share from the $1.7 trillion conventional meat and seafood industry. This shift will mitigate agriculture-related deforestation, biodiversity loss, antibiotic resistance, zoonotic disease outbreaks, and industrialised animal slaughter.
When will cultivated meat make it to market?
As of mid-2020, several leading cultivated meat companies are transitioning to pilot-scale facilities that will manufacture the first wave of commercialised products following regulatory approval. The Singapore Food Agency approved the world’s first cultivated meat product for sale in December 2020. Shortly thereafter, the 1880 restaurant in Singapore marked the historic first commercial sale of the approved cultivated chicken bite produced by California-based Eat Just.
Further scaling production to significantly larger facilities than what currently exists will require solving an array of complex challenges. These challenges range across four key areas: cell lines, cell culture media, bioprocess design, and scaffolding.
Solving these challenges and propelling the cultivated meat industry into maturity will require an influx of funding from both the public and private sectors. New courses, research centers, and training programmes for scientists, as well as policy work and regulatory action will accelerate progress.
The field will also need new companies, contributions from existing life science companies, and openness to collaboration from existing cultivated meat companies. Many new career opportunities will need to be filled by talented scientists, businesspeople, and other contributors along the value chain.
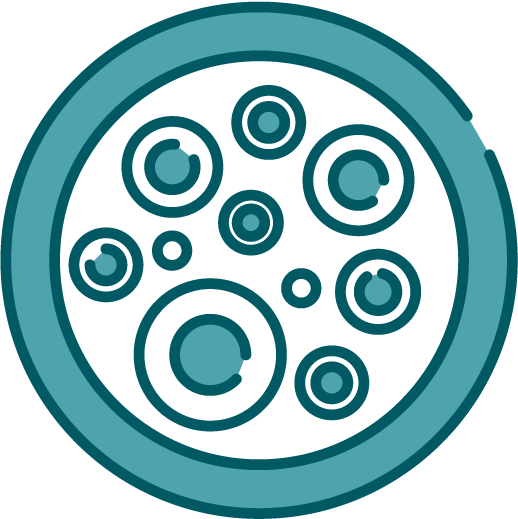
Cell lines
Many different cell types can be used to cultivate meat. Further research is needed to make cell lines more accessible and to determine how the selection of a cell type and its properties influence the downstream process considerations.
The current state of cell lines
Many cell types can be used to cultivate meat.
The starting inputs for cultivated meat are cells that can self-renew and differentiate, otherwise known as stem cells. Stem cells used for cultivated meat production can be acquired or derived in several ways. The most common method is taking a cell sample from a live animal. This can be done using minimally invasive methods.
Different types of stem cells, such as skeletal muscle stem cells (i.e., myosatellite cells), adipogenic stem cells, or mesenchymal stem cells, can be acquired in this way. Stem cells can also be obtained by taking a cell sample of specific organs to create other products. For example, cells from mammary glands can be used for milk production, and cells from livers for foie gras. In some cases, these cells may be acquired by biopsying a recently slaughtered animal where the tissue is still viable, which could be important for determining compliance to religious laws (e.g., halal, kosher).
Stem cells known as pluripotent stem cells can be acquired from an early-stage embryo. Cells such as fibroblasts or peripheral blood mononuclear cells can be reprogrammed into induced pluripotent stem cells. Those source cells are obtained from methods such as a cheek swab or blood draw. In all cases, the acquired cells originate from healthy animals alongside extensive documentation that ensure the quality and traceability of the cells.
Deriving cell lines requires many resources.
Currently, the majority of cultivated meat manufacturers work with well-characterised cell lines, which have the ability to continuously proliferate over time. Some producers may select primary cells, which by definition have a finite life span. Currently, access to cell lines from species used for cultivated meat production is the major barrier for new research endeavors.
The creation of new cell lines can be both time- and resource-intensive, often taking 6-18 months to derive and sufficiently characterise a single line. In medical research, the creation of large-scale cell line biorepositories is often nationally-sponsored, emphasising their fundamental importance in advancing research. Similar government-backed projects are needed in cultivated meat.
Increasing access to cell lines is a priority for the industry.
To accelerate cell line availability, GFI is funding multiple research projects that create cell lines from species spanning livestock, poultry, and aquatic animals. GFI has also partnered with reagent provider Kerafast to bank and distribute cell lines submitted by researchers from around the world in academia and industry. B2B companies specialising in cell line creation and distribution are pursuing similar strategies.
Additional efforts are needed to generate and characterise cell lines from more species and different cell types, create protocols for cell isolation and culturing conditions, and discover low-cost, animal-free cell culture medium formulations. Partnerships with conventional meat producers and aquaculture groups that often work with animal cell lines may accelerate R&D in these areas.
Cell line challenges
Cell types have specific pros and cons.
As described previously, there are many cell types that can be used as starting inputs for cultivated meat production. However, more research is needed to determine which cells will be best suited for large-scale manufacturing or the creation of specific product types. Intrinsic cell characteristics, such as suitability for suspension growth, doubling times, growth rates, metabolism, differentiation capacity, and genomic stability can vary between cell type and species.
These characteristics must be weighed alongside techno-economic models and bioprocess design considerations to inform cell line selection. However, this is challenging. Species used in cultivated meat production (especially aquatic animals) are much less researched than humans, mice, or hamsters, which have been the mainstay of the biomedical and biopharmaceutical industries.
Engineering cells can inform research and development.
Cell engineering can accelerate the development of cell lines suitable for cultivated meat. Engineering cell lines can dramatically improve the efficiency or productivity of the production process, or even influence end product attributes such as nutrition.
Cell line engineering can take place in the form of adaptation or genetic engineering. Adaptation involves the serial subculture of a cell line in varying conditions along with selection over time. This process yields a cell line adapted to a new set of conditions or exhibiting a new trait. Common forms of adaptation include adaptation to serum-free medium or suspension growth.
Genetic engineering entails permanent changes by either removing, rearranging, or introducing DNA. While some cultivated meat companies have stated they do not plan to use genetic engineering, several others have filed patents describing various engineering approaches. Ultimately, the extent of genetic engineering and types of modifications incorporated into final products will be dependent on the regulatory approval process for engineered products, as well as consumer perceptions in different regions.
What comes next for cell lines
Democratisation of cell lines will open new doors.
In the future, researchers will have easy access to many well-characterised cell lines housed in multiple biorepositories spread across the globe. To get there, more public funding is needed to attract experienced stem cell biologists who can apply their knowledge and tools to the cultivated meat field. Researchers and companies alike should be motivated and incentivised to deposit cell lines in public repositories to lower the burden of entry throughout the industry.
To help overcome challenges in cell line engineering, life science companies and contract research organisations that specialise in cell line development are needed. These companies can apply high-throughput techniques and automation technologies to improve cell line R&D and engineering efforts. Together, these efforts can support a more robust research ecosystem that will generate new findings, create new tools, reduce costs, and accelerate the pace of commercialisation.
Check out additional reading and resources
Discover papers, GFI resources, ongoing research projects, and research opportunities related to cell lines.
Featured article
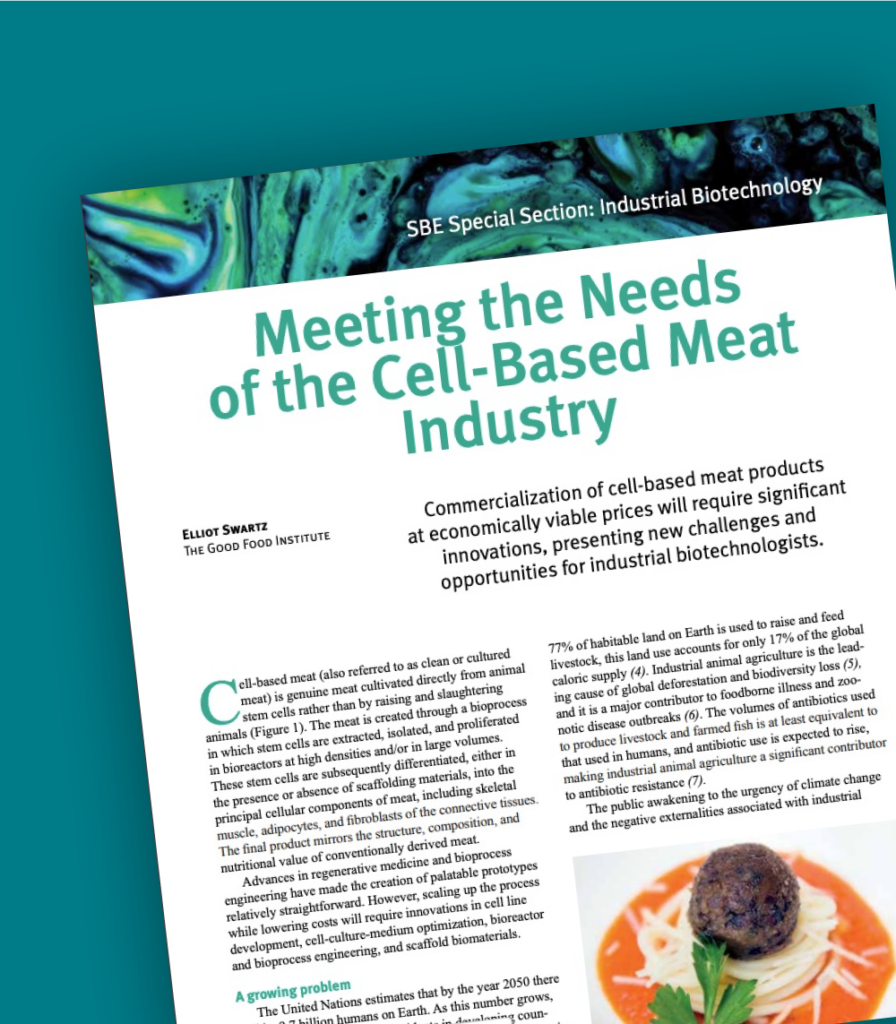
Read “Meeting the needs of the cell-based meat industry” published in the Chemical Engineering Process magazine
GFI-funded research projects
Learn about ongoing research by GFI grantees to accelerate cell line development for cultivated meat.
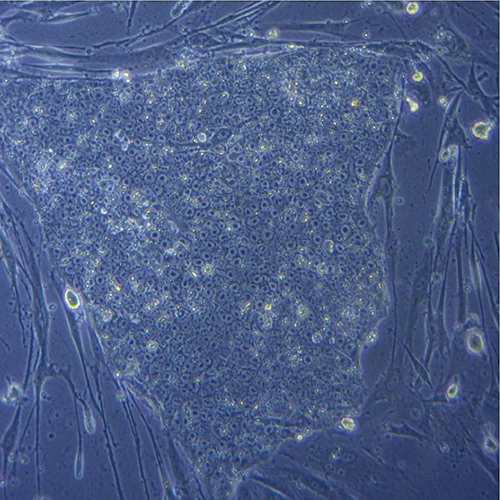
The Frozen Farmyard repository
Learn about Dr. Gareth Sullivan’s work to develop a “frozen farmyard” cell line repository for cultivated meat.
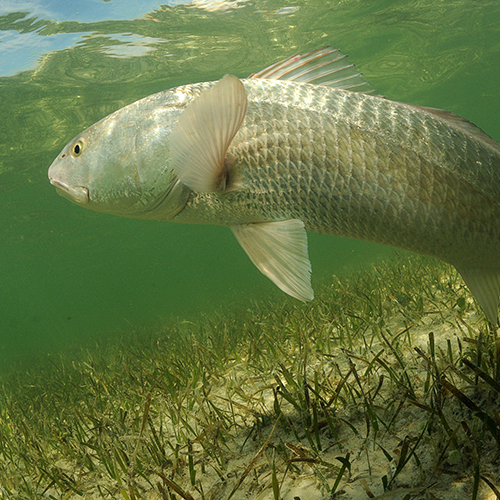
Seafood cell lines
Learn about Dr. Kevan Main and Dr. Cathy Walsh’s work at Mote Marine Laboratory to develop cell lines and methodology for cultivated seafood.
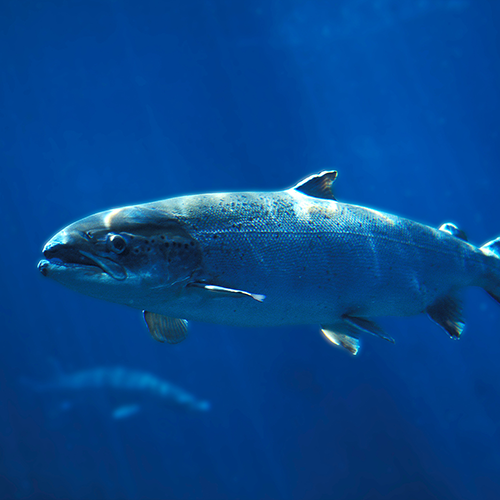
Myosatellite lines from Atlantic salmon
Through the GFI grant program, the Kaplan lab is developing myosatellite lines for cultivated Atlantic salmon at Tufts University.
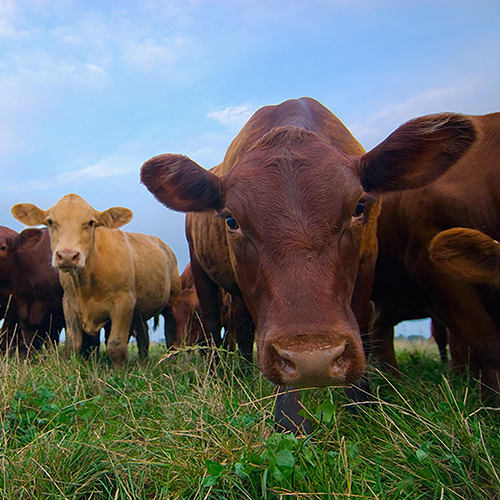
Making muscle cells
Learn about Dr. Ori Bar-Nur’s research to convert bovine and porcine fibroblasts into proliferative myogenic progenitor cells at ETH Zurich.
Research opportunities
Explore research opportunities in cell lines from our Advancing Solutions for Alternative Proteins database.
Cultivated seafood will need to be supplemented with long-chain omega-3 polyunsaturated fatty acids to be nutritionally equivalent or superior to conventional seafood. However, how these compounds can best be incorporated…
Both the cultivated meat industry and interested members of the general public would benefit from the creation of makerspaces focused on cultivated meat. These would be publicly available spaces where…
Cultivated meat research focuses primarily on muscle fibers and fat cells. However, other cell types serve functions that are often under appreciated in their relevance to cultivated meat. Co-culture methods…
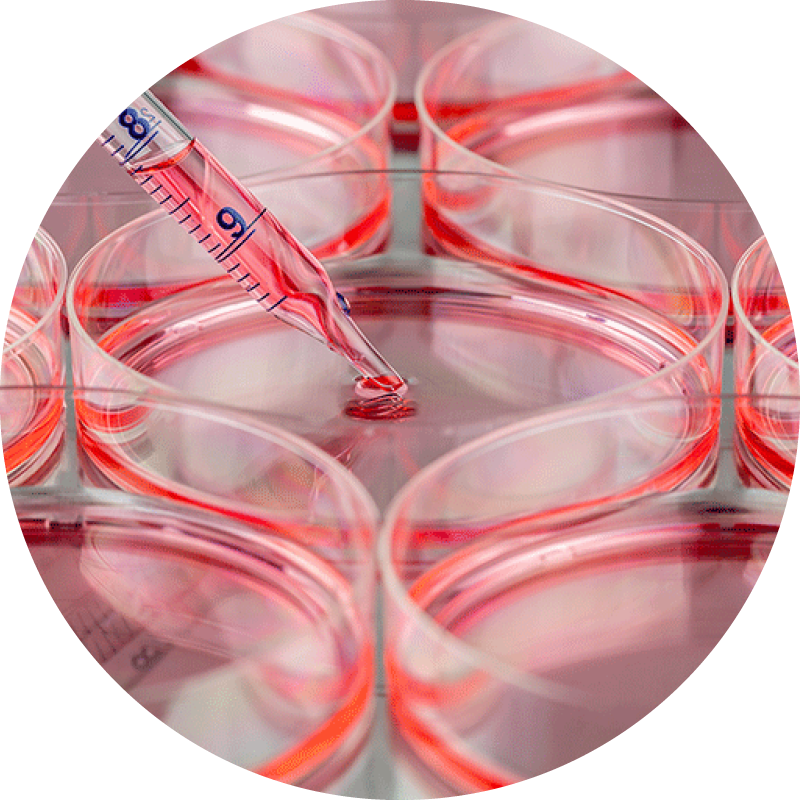
Cell culture media
The cell culture media contains the nutrients and growth factors that cells need to grow outside of the body. Research on optimised formulations, animal-free components, and recycling technologies are needed to make cell culture media significantly more affordable.
The current state of cell culture media
What’s in cell culture media?
The cell culture media is the most important technology underlying the near-term success of the cultivated meat industry. Cell culture media is composed of two groups of components tailored to a specific cell or species type.
The first group of components, called the basal media, provides essential nutrients. It typically consists of a buffered solution containing glucose, inorganic salts, water-soluble vitamins, and amino acids. The second is a group of specific added factors which permit the long-term maintenance, proliferation, or differentiation of cells. These added factors are often recombinant proteins, growth factors or hormones, and other ingredients such as lipids and antioxidants.
Animal serum will not be used in production.
While the basal media has persisted nearly unchanged since the 1950s, a transition away from other added factors originating from animal sources such as serum is still underway in many industries. The use of animal-derived components in cultivated meat production has prohibitive economic, food safety, and ethical constraints. Many companies have already publicly stated they are using medium formulations that are entirely animal-free, with many others likely to have already achieved this milestone.
The first approved cultivated meat product in Singapore was produced using fetal bovine serum (FBS), which is removed from the final product before consumption. The manufacturer Eat Just has stated that the next wave of future products would be manufactured using entirely plant-based ingredients, foregoing the use of FBS.
Cell culture media challenges
Significant cost reductions are possible without large technological leaps.
The largest challenge the cultivated meat industry faces is not simply foregoing animal components in the cell culture media but rather discovering how to do so affordably and how to optimise affordable formulations for maximised productivity.
Media cost reduction models have projected achievable prices of less than $0.25 per liter using existing technologies. This would amount to upwards of a 99.9 percent cost reduction versus media manufactured and sold to the biomedical and biopharmaceutical industries—a seemingly daunting task.
However, considerable progress has already been made in achieving these dramatic cost reductions. For instance, a team of researchers at Northwestern University demonstrated a popular stem cell medium formulation could be produced for 97 percent less versus its commercially-sold counterpart.
Mosa Meat, a leading cultivated meat company in the Netherlands, announced in July of 2020 that they had reduced their medium costs by 88 fold. The XPRIZE Foundation is currently seeking teams that will be awarded a $2M prize by developing an animal-free medium at the lowest cost.
Reducing costs of recombinant proteins is the largest price reduction lever.
Multiple options for cost reduction are being pursued across the industry. The most urgent action is to address the high costs of recombinant proteins and growth factors. While some B2B manufacturers are scaling the production of proteins and growth factors using microbes, fungi, or plants as expression systems, others are looking to replace these proteins and growth factors with plant-based alternatives.
Costs can also be reduced by sourcing media components at food or feed grade, reducing the overhead and operational costs associated with producing pharmaceutical-grade components. This means that it is likely that much of the glucose and amino acids—which together make up the bulk of the cellular biomass—will originate from abundant crops and readily-grown microbes.
Some cultivated meat companies have already struck partnerships with animal feed manufacturers to obtain these components at feed-grade, allowing them to tap into mature supply chains.
Demand forecasts can help to predict industry needs.
Many existing media manufacturers have the capacity to contribute to the cultivated meat industry, but data-driven projections of total volumes and demand forecasts for specific media components are needed to incentivise these manufacturers to mobilise the appropriate resources.
Challenges still remain in identifying the sources of upstream raw materials that are best suited for each species and cell type used by cultivated meat manufacturers. Several methods can be used to achieve desired performance results through formulation optimisation. However, publicly-available data on cell-specific formulations will be needed. These open data can be used for machine learning applications and to refine computationally-assisted algorithms for in silico experiments that save time and costs.
Life science companies with high-throughput and microfluidic capabilities can also lend services for formulation discovery. Importantly, the selection and production of these raw materials will also be influenced by how they fit into to-be-determined regulatory frameworks, their environmental sustainability footprints, and the social sustainability implications for the farmers and other workers along the value chain.
What’s next for cell culture media?
Long-term optimisations will contribute to further cost reduction and sustainability improvements.
There are many additional opportunities for cost reduction and optimised formulations that are likely to contribute to the commercial success of cultivated meat over longer time horizons. These include developing novel technologies for medium recycling, valorising waste streams or metabolites produced by cells, engineering growth factors with improved properties, and researching how cell culture media components influence the nutritional profiles of end products. Progress in each of these areas will require more public funding as well as co-developing solutions with participants from academia, the life science industry, and the cultivated meat industry.
Check out additional reading and resources
Discover papers, GFI resources, ongoing research projects, and research opportunities related to cell culture media.
GFI resources
Dig into open-access resources created by our experts.
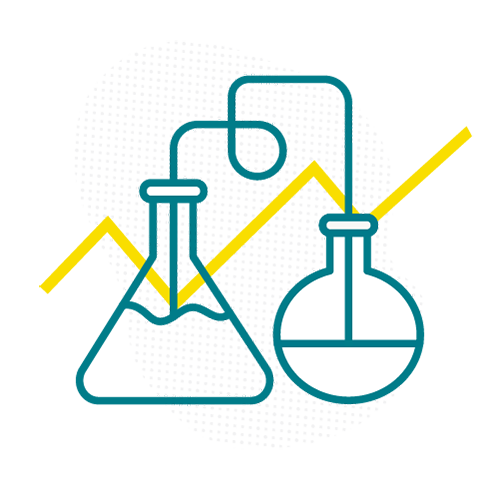
Cell culture media and growth factor trends in the cultivated meat industry
GFI and TurtleTree Scientific have partnered to distill a snapshot of current cultivated meat industry progress and needs, with an eye toward future demands and cost reduction prospects…
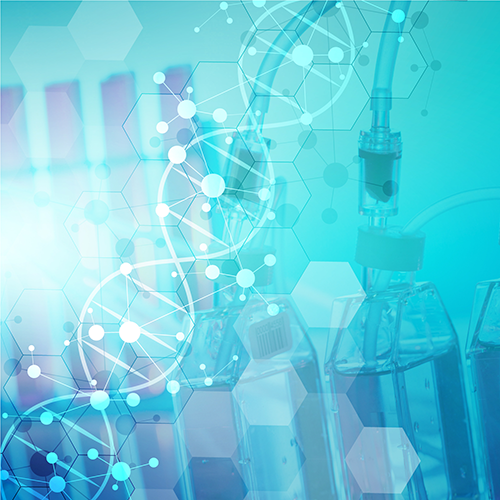
Analysing cell culture medium costs
This white paper explains different routes to lowering the cost of cell culture medium and making cultivated meat economically viable.
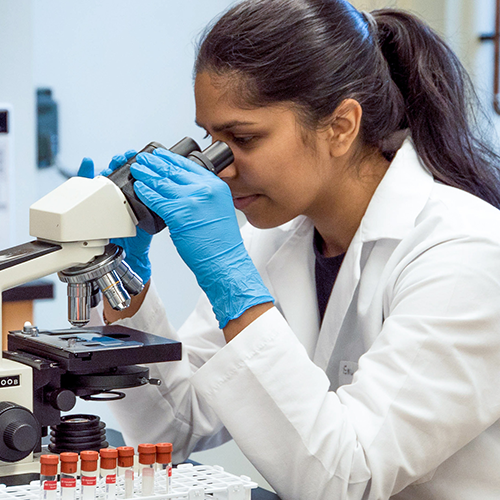
Cultivated meat research tools database
Use this crowdsourced directory to find species-specific information on research tools, reagents, protocols, and data for cultivated meat researchers.
GFI-funded research projects
Learn about ongoing research by GFI grantees to accelerate cell line development for cultivated meat.
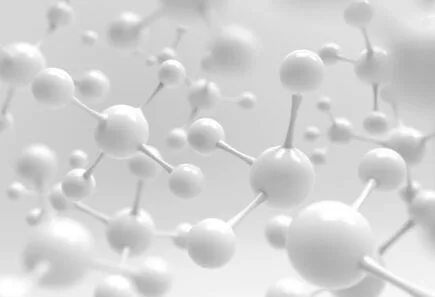
Formulating media with macromolecular crowding
Learn about Dr. Connon and Dr. Gouveia’s work at Newcastle University, UK to formulate growth media for cultivated meat with macromolecular crowding.
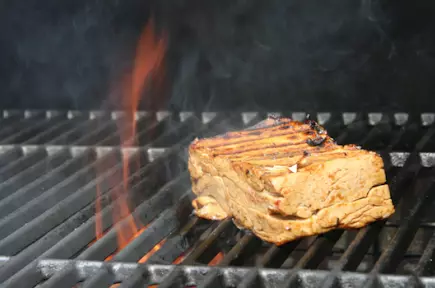
Optimising media for chicken cells
Learn about Dr. David Block’s work to perfect growth media for cultivated chicken at University of California, Davis.
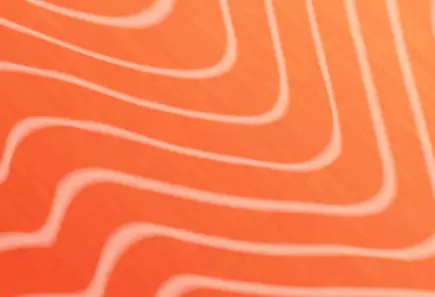
Machine learning for fish growth media
Learn about Dr. Reza Ovissipour’s research using machine learning to optimize growth media for fish cells at Virginia Tech.
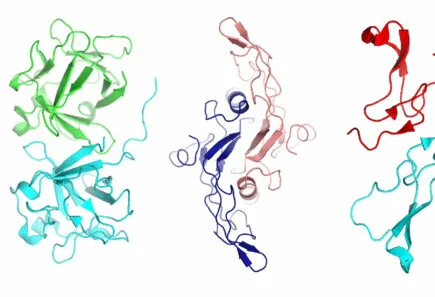
Lowering the cost of growth factors
Learn about Dr. Peter Stogio’s research engineering improved and lower-cost growth factors for cultivated meat at University of Toronto.
Research opportunities
Explore research opportunities in cell lines from our Advancing Solutions for Alternative Proteins database.
The identification of non-animal, non-recombinant proteins with similar functionality to serum albumin and transferrin will lead to major cost reductions in cell culture media development, facilitating progress toward achieving price…
Cultivated seafood will need to be supplemented with long-chain omega-3 polyunsaturated fatty acids to be nutritionally equivalent or superior to conventional seafood. However, how these compounds can best be incorporated…
There is a need for a supplier of low-cost growth factors produced without the use of animals to support the proliferation phase of cultivated meat production. The cost of growth…
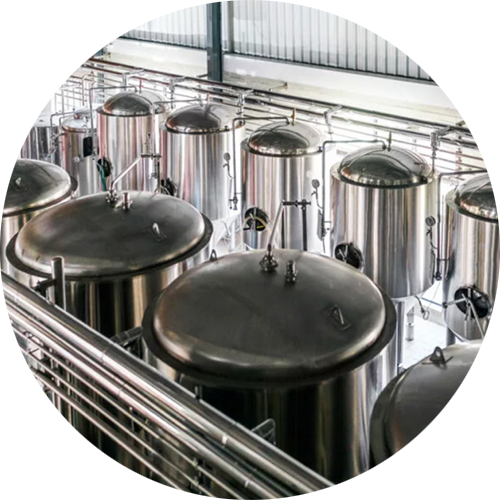
Bioprocess design
The bioprocess design holds the key to unlocking large-scale production of cultivated meat. Additional research is needed to determine the best-suited bioreactors for different cell types and products as well as how future facilities will be operated.
The current state of bioprocess design
Bioreactors permit large-scale cell cultivation.
The most important aspect of the bioprocess design is the bioreactor. Bioreactors (known colloquially as cultivators) provide the housing and control the conditions that enable cells to grow. For instance, bioreactors control the temperature, oxygen levels, and delivery of cell culture media.
They also enable monitoring of other important parameters such as metabolite levels, pH, and biomass accumulation. Different types of bioreactors have long-standing histories in other industries that rely on animal cell culture. Current models are being used by cultivated meat manufacturers at this time.
Innovations in bioreactor technology represent a large whitespace opportunity.
However, these current models of bioreactors are not necessarily optimised for cultivated meat production. This is because the bioreactor and overall bioprocess design are influenced by the selection of cell type and its properties (e.g., anchorage dependence), the cell culture media composition, and the overall goals of the process.
While some aspects of proliferation and differentiation overlap with other industries, cultivated meat manufacturers must optimise to perform those operations efficiently at very large scales and very low costs throughout the process. This suggests that novel bioreactor designs and modes of operation may be pursued by cultivated meat manufacturers and B2B suppliers.
Funding and consortia focused on bioreactors have begun to mobilise.
Discovering what aspects of existing bioreactors may be changed or how new bioreactors may be designed is an area of active research. In industry, several B2B companies aim to develop novel bioreactors for cultivated meat manufacturers, including those that operate continuously. Large life science companies such as Merck KGaA, Darmstadt, Germany, have sponsored research grants for novel designs.
Multiple companies and other stakeholders have formed the Cultivated Meat Modeling Consortium, which aims to apply computational modeling techniques to answer questions related to bioprocess design that may otherwise be overly resource- or time-intensive to pursue. Additional collaborations between cultivated meat manufacturers and life science companies or researchers experienced in fermentation and bioprocess scale-up may fare well in accelerating R&D in this area.
Bioprocess design challenges
Pilot-scale facilities represent the first major scale-up progress.
Scaling up a bioprocess generally takes place in three separate phases: lab-scale, pilot-scale, and demonstration or commercial scale. As cultivated meat companies move into their pilot-scale facilities, they will face the challenge of designing efficient, fit-for-purpose bioprocesses that can either scale up to sizes that dwarf existing animal cell culture facilities or scale out using processes and facility designs that are portable.
Insights into potential bottlenecks for these facilities can be gained by funding open-access techno-economic models and facility blueprints with an eye toward both regulatory and sustainability considerations. Once achieved, a menu of flexible financing options for future facilities will be needed. The formation of co-development manufacturing organisations (CDMOs) may also assist cultivated meat manufacturers by removing technical, financial, and capacity burdens.
Planning for success
Research into other aspects of the bioprocess design will also be important. Cultivated meat manufacturers will need to be equipped with state-of-the-art sensor equipment, ideally integrated into the bioreactors themselves. Companies will also need to develop processes that are amenable to automation and real-time responses. These processes should integrate medium recycling and waste stream valorisation to maximise their efficiencies.
The differentiation, maturation, and harvesting of cells for cultivated meat is likely to require unique innovations and bioreactor designs that address specific challenges for each of these phases. Success in these areas may be bolstered by interdisciplinary groups of biologists and engineers that have access to research centers equipped with pilot-scale capabilities that can serve as a testing ground for new technologies.
These research centers may be sponsored by collaborations between academic and biotechnology industry stakeholders (such as Planet B.io in The Netherlands and partnerships between The Technion, The Kitchen Hub food tech incubator, and Aleph Farms in Israel) or come from governmental grants.
The Singaporean government has moved quickly to build a burgeoning ecosystem, with millions invested into cultivated meat projects and industry incentives. In the United States, the National Science Foundation’s award to researchers at UC Davis will lay the foundation for a cultivated meat research center on campus over the next five years.
Further buy-in from governments around the world will be crucial for accelerating the commercialisation of cultivated meat.
What’s next for bioprocess design?
All hands on deck
The size and growth of the cultivated meat industry beyond the next decade will be largely determined by the implementation of innovative bioprocess designs that form the basis of facility design and their operation. Producing volumes that capture just one percent of the global meat market will require extensive new infrastructure that can be supported by governments, multinational corporations, meat and life science industry incumbents, and a diverse and interdisciplinary workforce.
A maximised positive impact on the planet will also depend on future facilities being co-located near renewable energy sources. Other important aspects include easy access to raw materials, job opportunities that are equitable across rural and urban economies, and product access that is not limited to wealthy nations.
Check out additional reading and resources
Discover papers, GFI resources, ongoing research projects, and research opportunities related to bioprocess design.
The Cultivated Meat Modeling Consortium (CMMC)
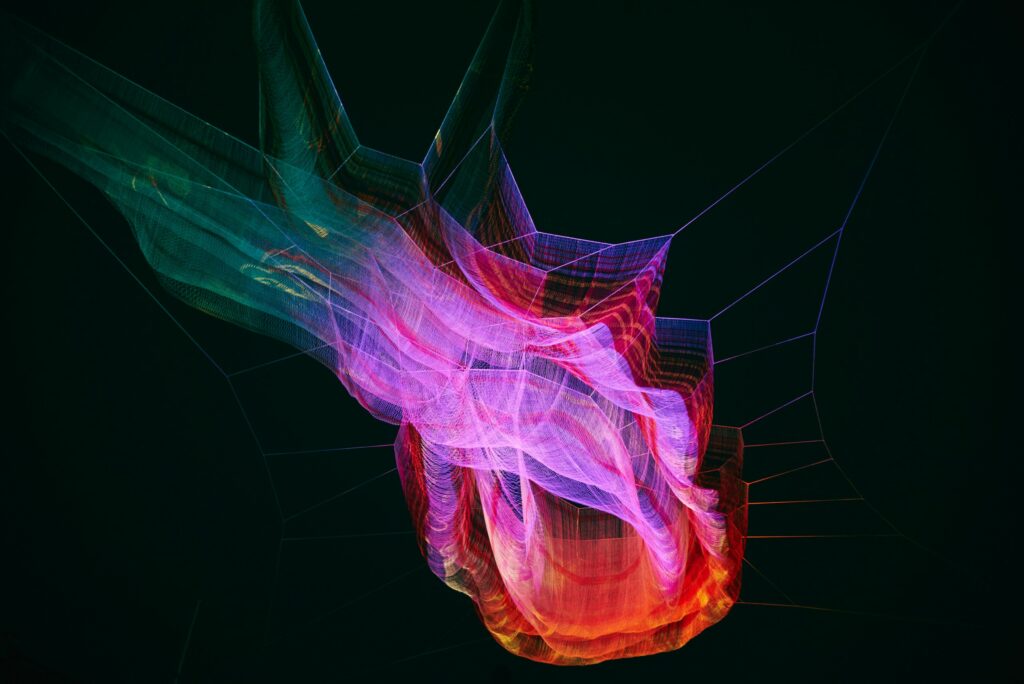
Founded by GFI grantee Dr. Simon Kahan, the CMMC is an international and interdisciplinary coalition working to advance cultivated meat through computational modeling.
Analysing cell culture medium costs
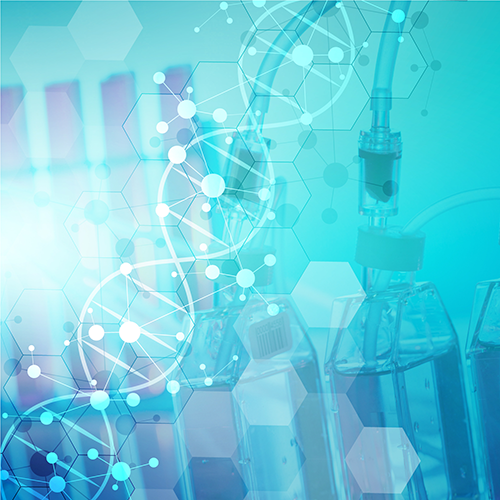
This white paper explains different routes to lowering the cost of cell culture medium and making cultivated meat economically viable.
GFI-funded research projects
Learn about ongoing research by GFI grantees to accelerate advances in bioprocess design for cultivated meat.
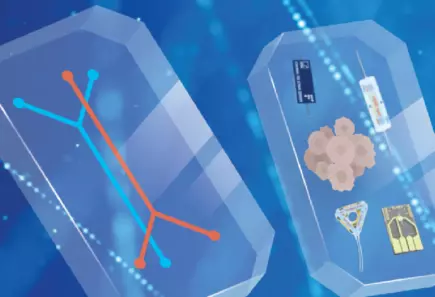
Integrating sensors into bioreactors
GFI grantees Dr. Ivana Gadjanski and Dr. Vasa Radonic are integrating sensors into bioreactors for cultivated meat production.
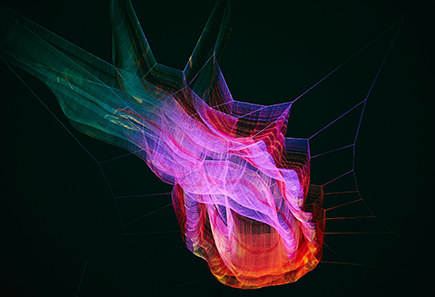
Computational modeling
GFI grantee Dr. Simon Kahan at the Cultivated Meat Modeling Consortium is using computational modeling to improve bioreactor design for meat cultivation.
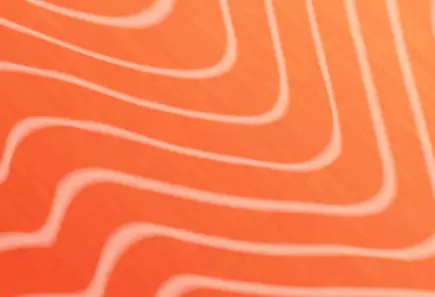
Machine learning for fish growth media
Learn about Dr. Reza Ovissipour’s research using machine learning to optimize growth media for fish cells at Virginia Tech.
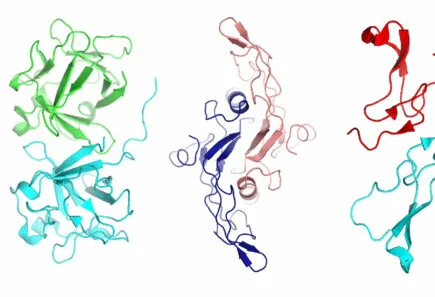
Lowering the cost of growth factors
Learn about Dr. Peter Stogios’ research engineering improved and lower-cost growth factors for cultivated meat at University of Toronto.
Research opportunities
Explore research opportunities in bioprocess design from our Advancing Solutions for Alternative Proteins database.
Cultivated seafood will need to Stretching of engineered muscle constructs has been previously demonstrated to induce alignment and maturation of muscle fibers, which is desirable for whole cut cultivated meat. Stretch stimuli could also be…
Both the cultivated meat Cultivated seafood will need to be supplemented with long-chain omega-3 polyunsaturated fatty acids to be nutritionally equivalent or superior to conventional seafood. However, how these compounds can best be incorporated…
The manufacturing capacity for rapid and cost-effective scale-up of alternative protein production is a current constraint on the growth of the industry. Repurposing and retrofitting stranded or underutilized assets such as shuttered…
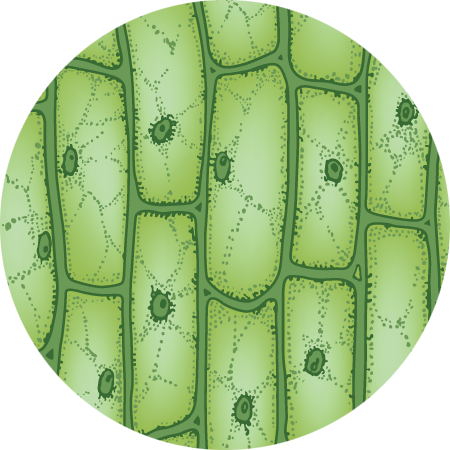
Scaffolding
Scaffolding provides structural support for cells to adhere, differentiate, and mature, making it crucial for the creation of structured meat products like steak. More research is needed to uncover the best materials and methods for constructing different types of cultivated meat products.
The current state of scaffolds
What do scaffolds do?
The development of scaffolds for cultivated meat requires expertise in cell biology and tissue engineering with a focus on the role of the extracellular matrix. Tissue engineers aim to recapitulate in vivo tissue growth outside of the body for both biomedical applications and cultivated meat.
To do so, scaffolds must provide access to oxygen and nutrients and have material properties (e.g., mechanical, biochemical) that are compatible with the cells housed within it. Scaffolds, together with the cell culture medium, can dictate how cell populations grow and differentiate. In some instances, scaffolds are used as microcarriers to aid the proliferation of anchorage-dependent cells in suspension bioreactors.
What properties do scaffolds have?
The most likely materials to be used for cultivated meat will be abundant, affordable, and food-safe. Some examples include polysaccharides such as chitosan, alginate, or cellulose; proteins such as zein; or complex composites such as lignin or textured vegetable protein.
The materials can be assembled by existing techniques including 3D printing, polymer spinning technologies such as electrospinning, decellularisation, tunable hydrogels, or even by nature itself (e.g., fungal mycelium). Several B2B companies, distinguished by their selection of material or method of assembly, aim to supply the industry with scaffolds. Many others are likely to be founded.
The selection of a scaffold and its properties will be highly dependent on the final product, with the scaffold having an increasingly important role in more structured products. Scaffolds may be intentionally designed to be biodegradable such that they are replaced with native extracellular matrix by the time a product is harvested. Alternatively, scaffolds can make up a significant portion of the final product, creating a hybrid product.
Scaffold materials that end up in a final product must meet requirements for how that product may be cooked and prepared, as well as how the material influences the product’s safety, digestibility, taste, and nutrition. Public databases of biomaterials and their properties can inform the selection of the most promising scaffold materials and methods to construct them.
Scaffold challenges
Structured products present numerous challenges.
The production of thick tissues remains challenging because all cells must lie within a short distance to nutrients and oxygen. There are two approaches to this challenge: a top-down approach where a prefabricated, porous scaffold becomes infused with cells, or a bottom-up approach where small, modular units of scaffolds and cells are constructed into a final shape.
Both approaches currently exist at small scales, with more research needed to understand the limitations of scaffolding technologies. These data will inform which scaffolds may be best suited for large-scale, commercial production of structured cuts of cultivated meat.
As previously described, the large-scale production of cultivated meat will rely on bioreactors. While microcarriers have been used extensively in other industries, understanding how different types of scaffolds may be incorporated into suspension or perfusion bioreactors is in its infancy. Computational modeling may assist in the selection of a scaffold’s material, shape, or topography, given certain fluid flows in different bioreactor models. Techniques to harvest scaffolds without damaging the cells or tissues will also be important.
Creative solutions are needed.
Other methods may use biomaterials to house cells in 3D microenvironments, providing them structural support but allowing self-organisation principles to govern their behavior within the material. These 3D microenvironments can be formed as spheres, tubes, or other shapes tailored to cell type, placed in bioreactors where they may have specific advantages (e.g., protection from shear stress), and constructed into complex shapes using bottom-up approaches. Similar outside-the-box strategies can contribute to our understanding of structured product creation.
What’s next for scaffolds?
Maximising potential
The holy grail of the cultivated meat industry is to produce a complex meat product such as a steak or chicken breast in a pre-programmed way, each and every time. However, current technologies cannot yet accomplish this feat. Significant advances in tissue engineering techniques will be needed to commercialise structured products at scale. Additional challenges lie in discovering the methods and materials that will be most amenable to large-scale production.
Check out additional reading and resources
Discover papers, GFI resources, ongoing research projects, and research opportunities related to cultivated meat scaffolds.
Featured paper
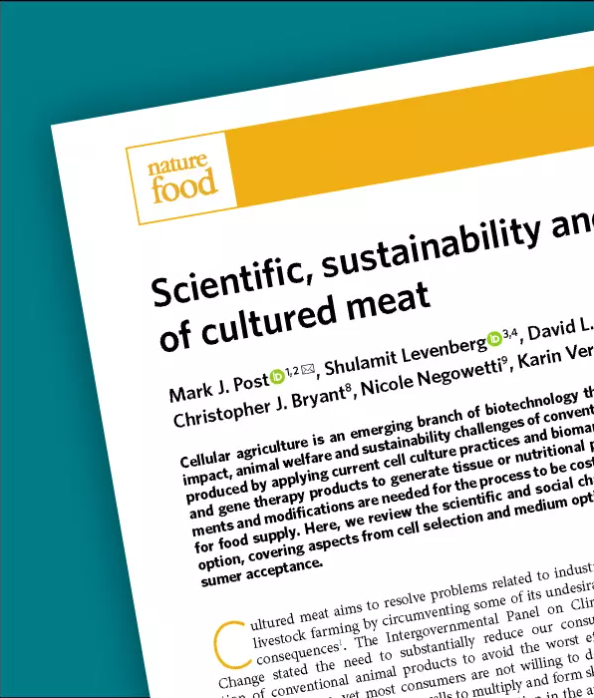
Read Dr. Mark Post’s paper “Scientific, sustainability and regulatory challenges of cultured meat” published in Nature Food.
GFI-funded research projects
Learn about ongoing research by GFI grantees to accelerate advances in cultivated meat scaffolds.
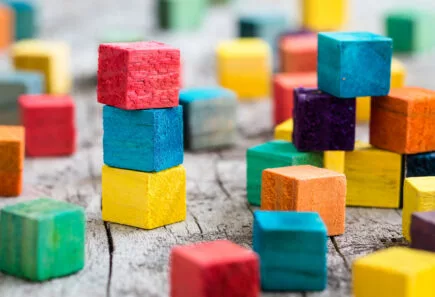
Cellular building blocks
Learn about Dr. Marcelle Machluf’s work designing cellular building blocks for cultivated meat with at Technion – Israel Institute of Technology.
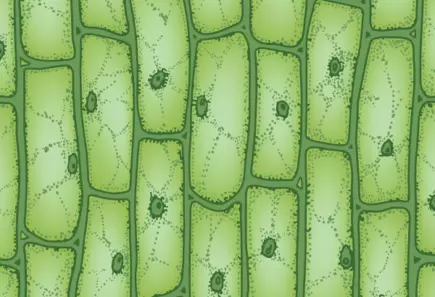
Plant-based scaffolds
GFI is building plant-based tissue scaffolds for cultivated meat with Dr. Masatoshi Suzuki at University of Wisconsin, Madison.
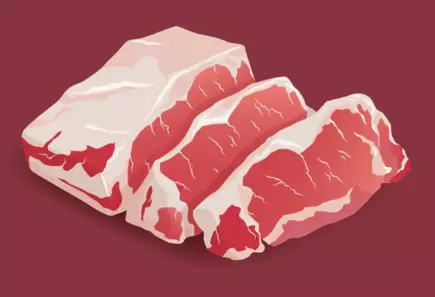
Developing marbled cultivated beef
GFI is developing marbled cultivated beef with Dr. Amy Rowat at University of California, Los Angeles.
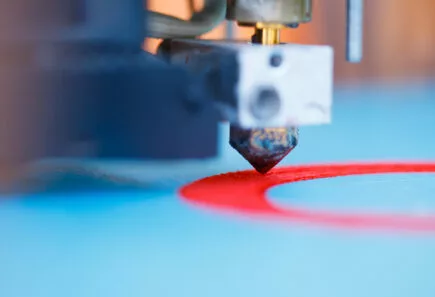
3-D printing bioinks
Learn about GFI grantee Dr. Sara Oliveira’s work for 3D bioprinting scaffolds for cultivated meat at the International Iberian Nanotechnology Laboratory in Portugal.
Research opportunities
Explore research opportunities in scaffolding from our Advancing Solutions for Alternative Proteins database.
Stretching of engineered muscle constructs has been previously demonstrated to induce alignment and maturation of muscle fibers, which is desirable for whole cut cultivated meat. Stretch stimuli could also be incorporated…
Cultivated seafood will need to be supplemented with long-chain omega-3 polyunsaturated fatty acids to be nutritionally equivalent or superior to conventional seafood. However, how these compounds can best be incorporated…
Both the cultivated meat industry and interested members of the general public would benefit from the creation of makerspaces focused on cultivated meat. These would be publicly available spaces where…
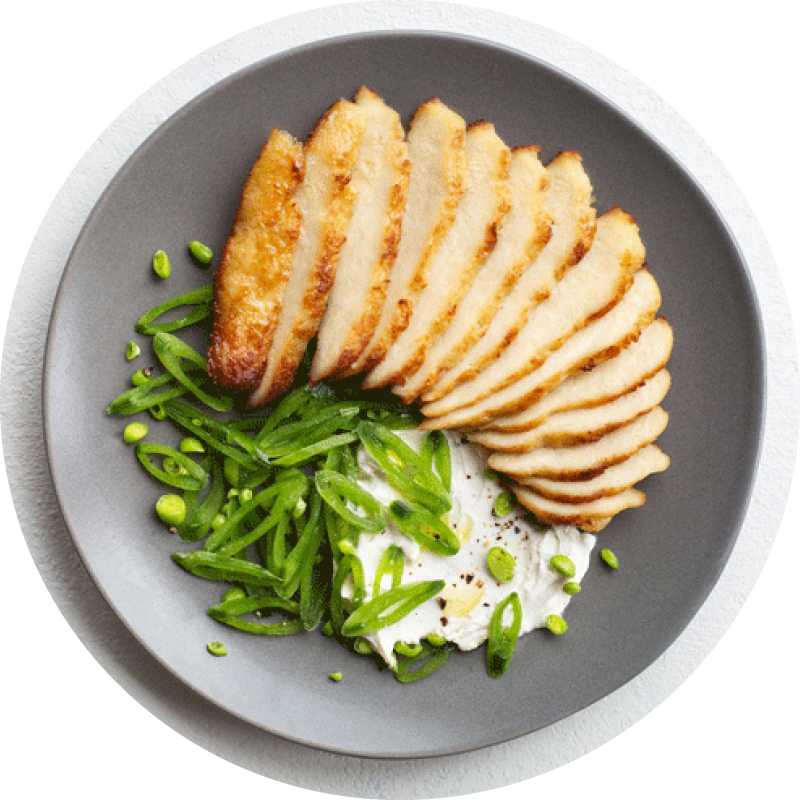
End product considerations
Some cultivated meat prototypes have been taste-tested but many sensory characteristics are unknown. Knowledge from meat science and food scientists can help create the full range of cultivated meat products that compete with or outperform their conventional counterparts on taste, quality, and nutrition.
The current state of end products
What might the first generation of cultivated meat products look like?
Many tasty, compelling cultivated meat prototypes have been created, including shrimp dumplings, pork sausages, chicken nuggets, beef steak strips, fish maw, foie gras, and salmon nigiri, to name a few. But key questions remain concerning what the first approved products will look like. Will they be structured or unstructured? What are their costs? And how will they be regulated?
The first generation of approved products may look quite different from company to company, but it’s likely we can predict some characteristics. From a technical standpoint, the creation of unstructured, minced product types are easier to create, thus this form is likely to represent the first generation of products on the market. As previously mentioned, fully structured products will be dependent on advances in scaffold technologies and are likely to arrive on the market later.
Similarly, the costs of first generation products will come at a premium. One way to offset these costs is by creating a hybrid product where cultivated animal cells are combined with plant-based ingredients. The percentages of each may vary depending on the product’s price, type, and taste profile. Some companies aim to sell cultivated animal fats as ingredients, while others may choose to focus on cultivated muscle tissues.
To be granted regulatory approval and be labeled as a meat product, cultivated meat must have similar nutritional profiles as their conventional counterparts. The nutritional data will have to be collected by companies and submitted to regulatory authorities prior to approval. To date, the nutritional data of prototypes remains privately held as companies continue to iterate on their first products. Sensory and nutritional data could be published in open-access journals to increase the transparency of the industry.
End product challenges
Competing on all aspects of meat quality
Replicating all of the features of conventional animal meat using cultivated meat processes is likely to be challenging. For instance, proteins and metabolites that give meat products their color, smell, and cooking properties may be expressed differently in cultivated meats.
Additionally, it is unknown whether the post-mortem enzymatic events that can influence meat texture occur similarly in cultivated meat products. Added ingredients that aid in the color, binding, and texture of cultivated meat may be needed, as featured in plant-based meats.
To discover the extent to which there are differences, meat scientists are needed to determine the properties of cultivated meat products. Additionally, food scientists will need to assist in formulating the best products across a range of versatile dishes. Research teams in academia that incorporate faculty from biological science departments together with meat and food science departments can accelerate progress in these areas.
What’s next for end products?
The future is bright.
Companies have a large toolset available to continue improving upon end-product characteristics. Improvements in nutrition may be possible by tailoring cell culture media ingredients for desired outcomes, such as increased omega-3 fatty acids or higher vitamin and mineral content.
Genetic engineering, if permissible, could be used to remove allergens or proteins linked to cancer risk in certain forms of meat. It could also insert genes that can fortify products with vitamin A precursors not found in conventional meats, or create personalised nutrition for specific populations.
Consumer research indicates that a majority of consumers are willing to try cultivated meat or even pay a premium. Willingness to try is highly correlated to the amount of information given to consumers about the cultivated meat production process and its benefits compared to conventional meats. Resources with accessible, educational information about cultivated meat, together with industry transparency, will be crucial for the adoption of the technology.
The Chicken, a test-kitchen and restaurant in Israel founded by SuperMeat in 2020 is intended to allow consumers to dine on cultivated chicken dishes made under the same roof. Novel dining experiences and new culinary creations promise to lure in new consumers and redefine how we view our relationship with meat products. Cultivated meat promises to be an ever-evolving industry for decades to come!
Check out additional reading and resources
Discover papers, GFI resources, ongoing research projects, and research opportunities related to end-product formulation.
Featured paper
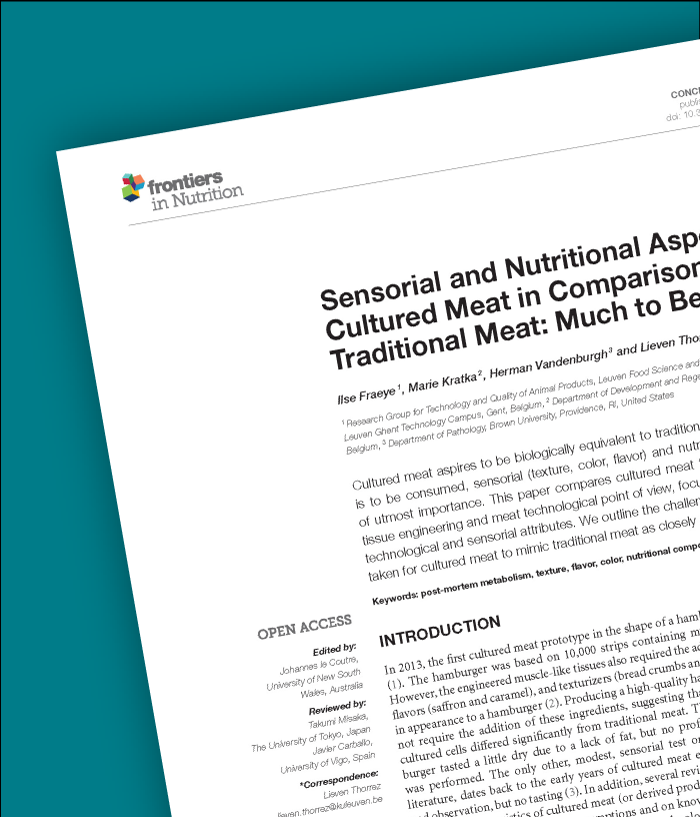
Read “Sensorial and Nutritional Aspects of Cultured Meat in Comparison to Traditional Meat: Much to Be Inferred” in Frontiers in Nutrition.
GFI resources
Dig into open-access resources created by our experts.
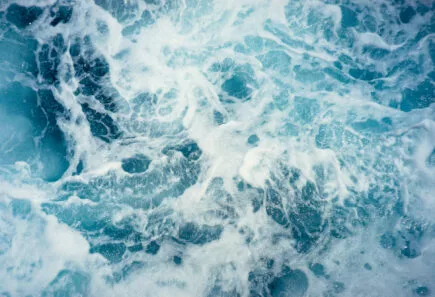
Aggregating data for alternative seafood
Use our open-access databases to explore how scientific taxonomies and evolutionary relationships map onto culinary categories of seafood.
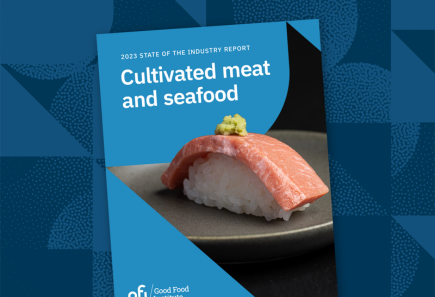
State of the Industry Report: Cultivated Meat
This global analysis of the cultivated meat industry covers investments, consumer insights, and scientific progress in this growing market sector.
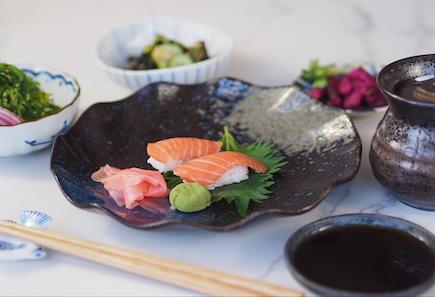
Deep dive: Cultivated meat end products
Learn about the science behind cultivated meat end-product design and characterisation, and the opportunities they present for innovation and open-access research.
GFI-funded research projects
Learn about ongoing research by GFI grantees to accelerate end-product formulation for cultivated meat.
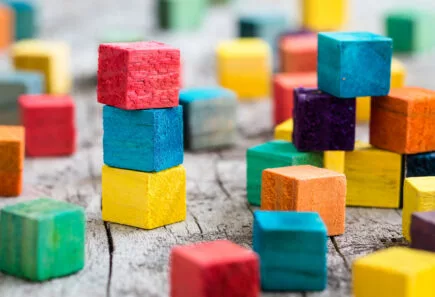
Cellular building blocks
Learn about Dr. Marcelle Machluf’s work designing cellular building blocks for cultivated meat with at Technion – Israel Institute of Technology.
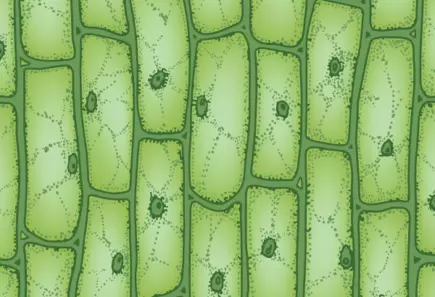
Plant-based scaffolds
GFI is building plant-based tissue scaffolds for cultivated meat with Dr. Masatoshi Suzuki at University of Wisconsin, Madison.
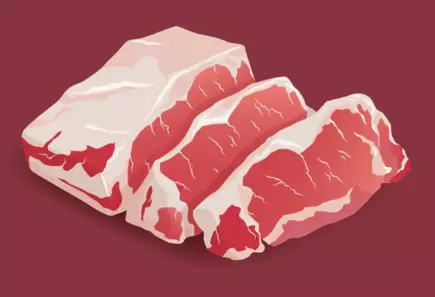
Developing marbled cultivated beef
GFI is developing marbled cultivated beef with Dr. Amy Rowat at University of California, Los Angeles.
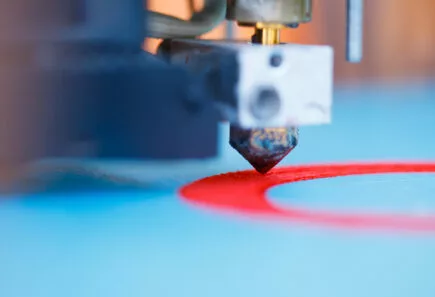
3-D printing bioinks
Learn about GFI grantee Dr. Sara Oliveira’s work for 3D bioprinting scaffolds for cultivated meat at the International Iberian Nanotechnology Laboratory in Portugal.
Research opportunities
Explore research opportunities in scaffolding from our Advancing Solutions for Alternative Proteins database.
Cultivated seafood will need to be supplemented with long-chain omega-3 polyunsaturated fatty acids to be nutritionally equivalent or superior to conventional seafood. However, how these compounds can best be incorporated…
The manufacturing capacity for rapid and cost-effective scale-up of alternative protein production is a current constraint on the growth of the industry. Repurposing and retrofitting stranded or underutilized assets such as shuttered…
Enabling easy animal ingredient substitutions in a wide range of food products.